Understanding Carbon Capture and Storage Technologies
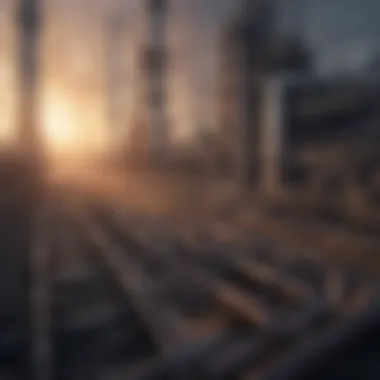
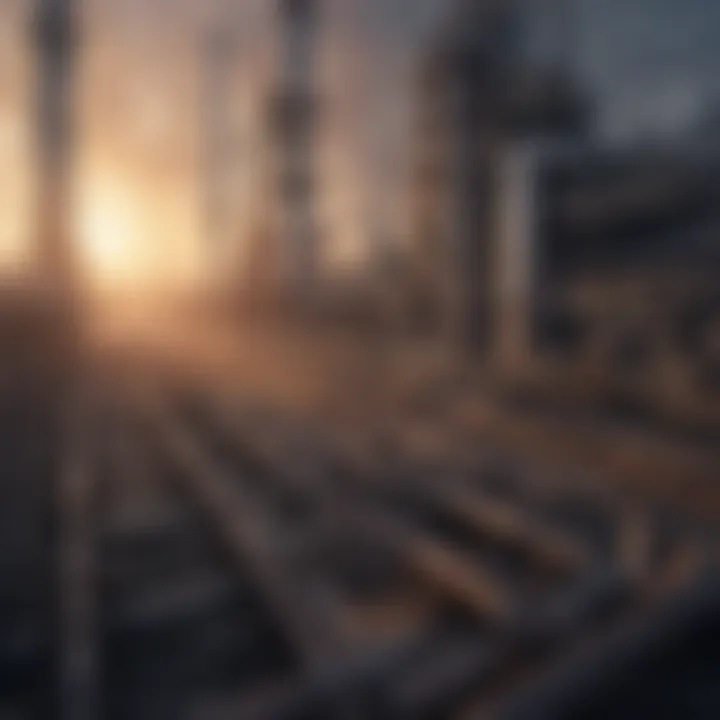
Intro
The increasing urgency surrounding climate change has prompted a closer examination of technologies capable of mitigating greenhouse gas emissions. Among these, Carbon Capture and Storage (CCS) stands out as a pivotal approach. This article seeks to unpack the complexities of CCS, elucidating its principles, methodologies, and the broader implications for environmental management. The necessity for effective carbon management is clear, as rising atmospheric carbon dioxide levels pose significant threats to global ecosystems.
CCS encompasses three main stages: capturing carbon dioxide produced from power plants and industrial processes, transporting it to a storage site, and securely storing it underground to prevent its release into the atmosphere. By thoroughly exploring these components, this piece aims to inform on the capabilities, limitations, and future directions of CCS technologies.
Research Highlights
Key Findings
CCS technologies are advancing rapidly, with research revealing several important points:
- Current capture methods enhance the efficiency of existing power plants and industrial facilities.
- Cost-effective solutions are emerging, reducing the economic barriers to implementation.
- Vast geological formations are identified globally, suitable for safe CO2 storage.
- Comprehensive studies indicate that CCS can significantly reduce net emissions, particularly in hard-to-abate sectors like cement and steel production.
These findings suggest that CCS is not merely a supplementary solution but a crucial component of a multi-faceted strategy against climate change.
Implications and Applications
The implications of successful CCS deployment are substantial.
- Environmental Restoration: Implementing CCS can help restore balance to ecosystems disrupted by fossil fuel reliance.
- Regulatory Support: Many governments are investigating CCS as part of their climate action plans, potentially leading to supportive legislation and funding.
- Economic Opportunities: Developing CCS technologies presents new markets and job opportunities, particularly for engineers and technicians trained in environmental sciences.
- Global Cooperation: Climate change is a worldwide issue, and progress in CCS can foster international collaborations for environmental protection solutions.
Methodology Overview
Research Design
Research in the field of CCS employs a combination of theoretical and empirical approaches. Studies often focus on:
- Modeling Future Scenarios: Predicting the impact of widespread CCS adoption on global emissions.
- Case Studies: Documenting existing CCS projects to extract practical insights and best practices.
- Comparative Analyses: Evaluating different capture methods' efficiencies and costs.
Experimental Procedures
Experimental procedures vary based on the specific aspect of CCS being studied, but common approaches include:
- Laboratory Tests: Conducting trials on CO2 capture technologies in controlled settings to assess performance.
- Field Trials: Implementing pilot projects in real-world environments to gauge the effectiveness of CCS systems.
- Long-term Monitoring: Assessing the stability and security of CO2 storage sites over extended periods.
In summary, this article aims to provide a thorough exploration of Carbon Capture and Storage, offering insights into its potential as a viable solution for combating climate change while addressing the practicalities of its implementation and future outlook.
Prologue to Carbon Capture and Storage
Carbon Capture and Storage (CCS) is an essential technology in the quest to mitigate climate change. Understanding CCS involves grasping its mechanisms, significance, and challenges it faces in implementation. This section sets the stage for a comprehensive analysis of how CCS functions, why it is necessary, and what makes it a key player in reducing greenhouse gas emissions.
Definition and Importance of CCS
Carbon Capture and Storage refers to the processes designed to capture carbon dioxide emissions at their source, followed by transporting and storing it to prevent its release into the atmosphere. The importance of CCS lies in its ability to significantly lower the amount of CO2 that contributes to global warming. As industries, particularly energy production, are among the largest sources of these emissions, effective CCS technology can play a role in decoupling economic growth from greenhouse gas emissions.
The benefits of CCS go beyond mere emission reduction. First, it enables continuing the use of fossil fuels while transitioning to renewable energy sources. Second, it opens potential revenue streams through enhanced oil recovery, where captured CO2 is used to extract oil from depleted reservoirs. Furthermore, CCS supports the longevity of existing infrastructure while creating pathways towards a sustainable energy future.
The Science Behind Carbon Emissions
To comprehend the necessity for CCS, one must first understand the science of carbon emissions. Carbon dioxide is a greenhouse gas that traps heat within the Earth's atmosphere. The combustion of fossil fuels—found in electricity generation, transport, and industrial processes—releases unmanageable quantities of CO2. This increase in atmospheric CO2 levels is associated with changes in climate patterns, leading to severe weather events and temperature rises.
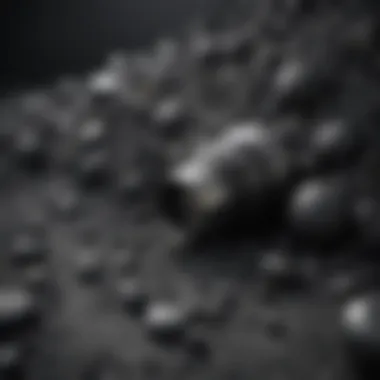
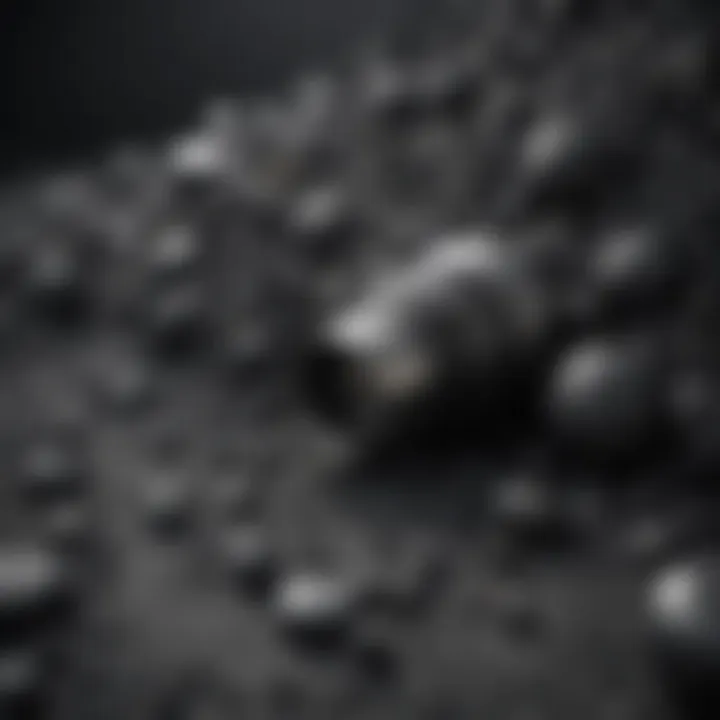
Grasping the scientific principles of carbon emissions highlights the urgency of CCS technologies. The solution lies not only in reducing emissions but also in actively removing and storing CO2. Research shows that emissions must be cut drastically to meet global temperature goals. Without CCS, achieving these targets would be near impossible. Therefore, CCS stands as a pivotal mechanism in climate change mitigation strategies.
Mechanisms of Carbon Capture
The section on mechanisms of carbon capture plays a crucial role in understanding how CCS technology works effectively to mitigate climate change. These mechanisms are designed to capture carbon dioxide emissions at source, preventing them from entering the atmosphere. Understanding these techniques is vital for assessing their effectiveness and feasibility in various industrial settings. Each method has unique characteristics, benefits, and challenges that need careful consideration.
Pre-combustion Capture Techniques
Pre-combustion capture techniques involve removing carbon dioxide before fossil fuels are burned. This method typically occurs in two stages: gasification and shift reaction. In gasification, solid fuels like coal are converted into a gas, primarily hydrogen and carbon monoxide. Then, during the shift reaction, the carbon monoxide reacts with steam to produce additional hydrogen and carbon dioxide.
This technique is effective because it allows for a purer hydrogen production, which can be used in energy generation with lower emissions. There are several advantages to pre-combustion capture:
- Higher capture efficiency due to the concentrated CO2 stream.
- Potential for hydrogen production, supporting a shift to cleaner energy sources.
However, it also poses some challenges, including high installation costs and the need for new infrastructure to support hydrogen use.
Post-combustion Capture Methods
Post-combustion capture methods focus on removing carbon dioxide from flue gases after fossil fuels have been combusted. The most common techniques in this category are absorption, adsorption, and membrane separation. Absorption involves chemical solvents, often amines, which chemically react with CO2, allowing it to be separated. Several studies show that this method can effectively remove up to 90% of CO2 emissions.
Advantages of post-combustion methods include:
- Compatibility with existing power plants and processes.
- Easier implementation without requiring major changes to combustion units.
Nonetheless, there are drawbacks, such as the high energy requirements for regenerating the solvents used, which can lead to increased operational costs.
Oxy-fuel Combustion Processes
Oxy-fuel combustion is a technique that burns fuel in an atmosphere rich in oxygen instead of air. This method generates a flue gas that is predominantly carbon dioxide and water vapor, making it easier to separate the CO2. The process involves significant modifications to existing boiler designs but holds promise for enhancing efficiency in carbon dioxide capture.
The main benefits of oxy-fuel combustion are:
- High purity of captured CO2, reducing the complexity of separation processes.
- The potential for higher efficiency in power generation with lower emissions.
However, the challenges include the need for oxygen production, which can be energy-intensive and costly.
Oxy-fuel combustion can provide a more straightforward path for carbon capture, but its feasibility in widespread application requires further exploration and development.
In summary, these mechanisms each present unique opportunities and limitations for carbon capture. Their understanding is the foundation for evaluating how CCS technology can be effectively integrated into current and future energy systems.
Transporting Captured CO2
Transporting captured carbon dioxide (CO2) is a crucial phase in the process of carbon capture and storage (CCS). The efficiency of CCS relies not only on capturing emissions but also on the effective movement of CO2 from the capture site to storage locations. This step bears significant implications for the overall success and viability of CCS initiatives. Understanding the various methods and infrastructures used for transporting CO2 is essential for evaluating the potential impact of these technologies on climate change mitigation.
Pipelines as Transport Infrastructure
Pipelines form the backbone of CO2 transportation in most CCS projects. They are designed to safely transport compressed CO2 over long distances. This method is mainly favored due to its economic advantages and efficiency. Pipelines can move large volumes of CO2 from industrial sources, such as power plants or factories, directly to storage sites.
The design of CO2 pipelines requires specific considerations. CO2 must be compressed to reduce its volume before moving through the pipeline. This process ensures that even vast quantities are moved efficiently. Additionally, the material used for pipelines must withstand the pressure associated with transporting CO2, as well as the potential for phase changes depending on environmental conditions.
One notable project employing CO2 pipelines is the Gorgon Project in Australia. This project aims to transport CO2 from a gas processing facility to an offshore storage location. The extensive network of pipelines used here exemplifies how large-scale operations can be successfully implemented.
Alternative Transport Methods
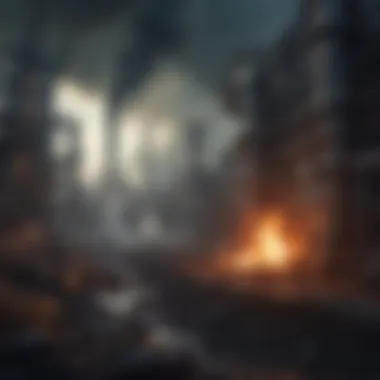
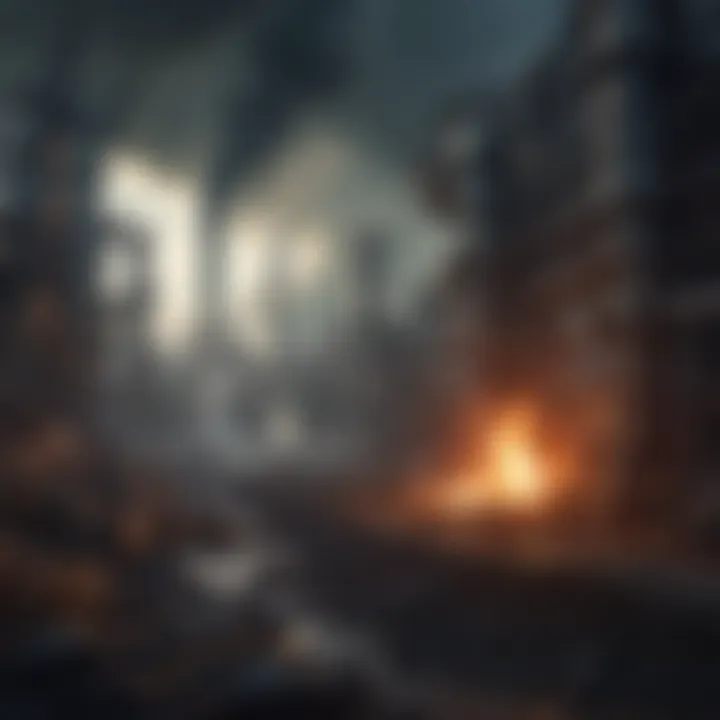
While pipelines are the dominant method for transporting CO2, other transport methods exist as well. These alternatives may be more suitable in specific scenarios. Some of the notable methods include:
- Ships: For locations not served by pipelines, ships offer a viable transport solution. CO2 can be liquefied and loaded onto specialized vessels, providing flexibility in reaching storage sites.
- Trucks: Smaller amounts of CO2 can be transported by tank trucks. This method is often used when distances are short, or pipeline infrastructure is not available.
- Rail: Rail transport is another alternative, especially for regions with developed rail networks. Tank cars can be adapted to carry compressed CO2.
Each of these methods presents unique challenges and benefits. For instance, ships can handle large volumes of CO2 and provide accessibility to offshore sites. However, they may incur higher logistical costs and require extensive port infrastructure.
Ultimately, the choice of transportation method will depend on various factors including distance, volume of CO2, and geographical considerations. Efficiency in transport is paramount, as delays or complications can detract from the overall effectiveness of CCS.
"The transportation of captured CO2 is not just a logistical concern, but a vital component in the strategy to manage and reduce carbon emissions effectively."
Understanding the nuances of transporting CO2 is key for industries and governments aiming to implement CCS solutions successfully. The right methods not only enhance operational efficiency but also play a critical role in ensuring that captured CO2 is sequestered safely and effectively.
Storage Solutions for Captured CO2
The significance of storage solutions in Carbon Capture and Storage (CCS) is paramount in the efforts to mitigate climate change. Once carbon dioxide (CO2) is captured, effectively storing it ensures that this gas does not re-enter the atmosphere, allowing for a meaningful reduction in greenhouse gas levels. There are several methods of storage, each with unique benefits and considerations. Understanding these options is essential for developing a comprehensive CCS strategy.
Geological Storage Approaches
Geological storage involves injecting captured CO2 deep underground, where it can be securely contained within rock formations. Suitable geological structures include depleted oil and gas fields, deep saline formations, and unminable coal seams.
The benefits of geological storage are substantial. It has the potential to store vast quantities of CO2 over long periods. Current estimates suggest that global geological formations could hold enough CO2 to offset many decades of emissions from fossil fuel use. However, careful site selection and monitoring are critical to ensure the integrity of these storage sites, as well as to prevent leakage.
Deep Ocean Storage Techniques
Deep ocean storage includes the direct injection of CO2 into deep sea water, where it can be isolated from the atmosphere for prolonged periods. The ocean's vast volume and pressure levels at great depths can assist in maintaining CO2 in a liquid state.
One of the primary advantages of this method is that it can potentially mitigate the risks of high-level emissions in coastal regions. Nevertheless, ecological and biological implications of ocean storage must be thoroughly researched. Concerns include impacts on marine life and potential changes in ocean chemistry.
Mineralization and Carbon Utilization
Mineralization refers to the process where CO2 is chemically reacted with natural minerals to form stable carbonates. This method not only offers a long-term storage solution but can also enhance economic opportunities by using CO2 in the production of building materials, such as concrete.
Carbon utilization initiatives are gaining traction as they combine the concepts of storage and beneficial use. By converting CO2 into valuable products, there is potential for developing a sustainable circular economy. However, this route requires extensive research and investment to ensure processes are efficient and commercially viable.
"Implementing effective storage solutions is as critical as capturing CO2. Each option has its effects on our environment and opportunities for innovation."
Environmental Implications of CCS
The environmental implications of Carbon Capture and Storage (CCS) are crucial to understanding the technology's role in addressing climate change. CCS is not just a technical solution; it also has extensive effects on ecosystems, public health, and commercial activities. As nations explore paths to reduce their carbon footprint, the environmental impacts of CCS become essential for assessing the feasibility and sustainability of these initiatives.
Potential Risks and Challenges
While CCS offers significant benefits, it does come with certain risks and challenges that require careful consideration. Some of the main issues include:
- Leakage Risks: One primary concern is the potential risk of CO2 leakage from storage sites. If captured CO2 escapes back into the atmosphere, it negates the benefits of the entire CCS process.
- Environmental Degradation: The construction and operation of CCS facilities can impact local ecosystems. Activities such as drilling and pipeline installation can disturb habitats, potentially harming wildlife.
- Long-term Monitoring: Ensuring the safety of CO2 storage sites calls for rigorous long-term monitoring. This adds complexity and cost to CCS initiatives, as continuous surveillance is necessary to detect any leaks or geological changes.
- Public Perception: Public acceptance can also pose challenges. Communities may be skeptical or resistant to having CCS facilities or pipelines near their homes, given the concerns about safety and environmental integrity.
"Understanding the potential risks associated with CCS is vital to designing robust safety measures and securing public trust in these technologies."
Regulatory and Policy Frameworks
The regulatory landscape around CCS is rapidly evolving, shaped by growing environmental concerns and climate policies. Effective governance can drive CCS deployment while ensuring environmental protection.
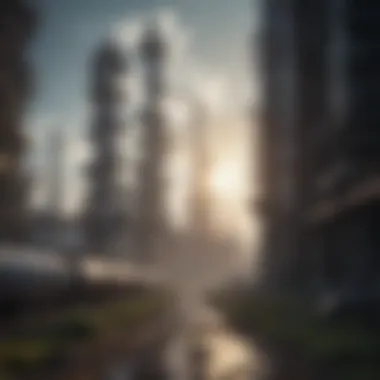
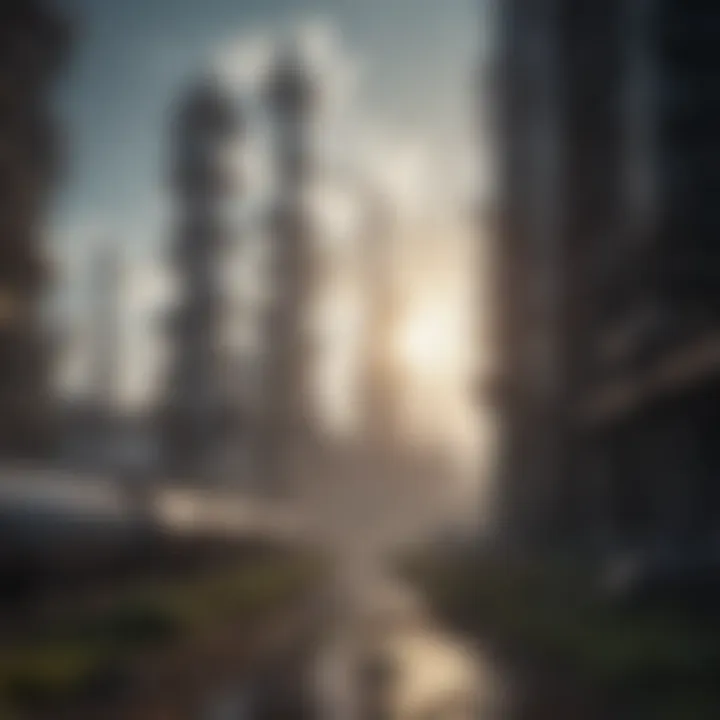
- Permitting Processes: Developing clear and streamlined permitting processes for CCS projects is essential. Governments need to establish regulatory frameworks that facilitate project approvals without compromising environmental safeguards.
- Incentives and Subsidies: Financial incentives are crucial for stimulating investments in CCS technology. Government programs that provide tax credits or grants can significantly lower the financial barriers for companies willing to invest in CCS.
- International Cooperation: Given the global nature of climate change, international cooperation is necessary for sharing best practices and regulatory policies. Countries can benefit from partnerships that align their CCS policies with global climate goals.
- Public Engagement: Educating communities about CCS technology and involving stakeholders in decision-making processes are vital. Transparency can foster public trust and acceptance of CCS initiatives.
In summary, the environmental implications of CCS encompass a range of factors that can either enhance or hinder its potential. Understanding and addressing the risks and regulatory frameworks surrounding CCS is essential for successful implementation.
Economic Aspects of CCS Implementation
The economic aspects of Carbon Capture and Storage (CCS) implementation are crucial for understanding its viability and effectiveness. Investing in CCS technology can have far-reaching consequences for climate change mitigation and securing long-term environmental goals. This analysis encompasses cost implications, financing strategies, and the overall economic impact of CCS initiatives. Examining these elements is essential for stakeholders, including governments, companies, and researchers, to grasp the full scope of CCS's role in the future of energy and emissions reduction.
Cost Analysis of CCS Projects
Cost analysis serves as a foundational aspect in engineering economically feasible CCS projects. Several factors contribute to the costs, including capturing CO2, transporting it, and storage solutions. In general, the costs can be broken down into three main categories:
- Capture Costs: This includes technology investment and installation needed to capture carbon from industrial processes or energy production.
- Transportation Costs: These costs arise from the infrastructure necessary to transport the captured CO2, usually via pipelines.
- Storage Costs: This involves securing suitable geological formations or other storage solutions to ensure safe and effective CO2 storage.
Significant research and development investments can lower costs over time. Breakthroughs in technology can enhance efficiency, thus reducing the overall footprint of the investment.
Apart from upfront costs, long-term operational and maintenance expenses also play a role. Organizations must assess the levelized cost of captured carbon (LCOC) to compare with alternative emissions reduction technologies.
Financing CCS Initiatives
Financing CCS initiatives poses its own set of challenges and opportunities. Innovative financing mechanisms are vital for encouraging private sector participation and investment in CCS technology. Several approaches can be explored:
- Public-Private Partnerships (PPPs): These collaborations can pool resources and share risks, allowing for larger-scale project implementation.
- Government Grants and Subsidies: Financial support from governments can stimulate early adoption of CCS technologies. This support can mitigate the financial burden on companies involved in these initiatives.
- Carbon Credits: Companies can generate revenue through Carbon Credits from capturing and storing emissions, making such initiatives financially attractive in a carbon-pricing environment.
- Venture Capital Investment: Investment firms actively seeking sustainable solutions may provide funding to promising CCS startups, accelerating early-phase growth.
Assessing these financing mechanisms is essential for institutions and firms contemplating CCS projects. They must not only focus on initial costs but also consider the overall financial sustainability of adopting such technologies. By prioritizing economic viability, CCS has the potential to be integrated more seamlessly into current energy systems.
Future Outlook for Carbon Capture Technologies
The future of Carbon Capture and Storage (CCS) technologies is a critical aspect of ongoing climate change mitigation efforts. The increasing urgency to address rising atmospheric CO2 levels makes it essential to explore where CCS is headed. The effectiveness of these technologies heavily influences global warming trends and policy decisions. As we look ahead, key elements can define the trajectory of CCS, including continued research innovations, international cooperation, and financial mechanisms to support its implementation.
Innovations in CCS Research
Research is a driving force in the advancement of CCS technologies. Various studies are focus on improving the efficiency and cost-effectiveness of carbon capture methods. This involves refining existing approaches and developing new materials for capturing CO2. Recent advancements in solvents used for absorption have made the process less energy-intensive. For example, new amine-based solvents show promise by providing higher absorption rates and easier regeneration.
Moreover, the use of membrane technology is gaining attention. Membranes can selectively allow CO2 to pass while blocking other gases, potentially lowering costs and energy requirements. Experiments with nanoporous materials also open doors for better capture at lower pressure, which can be significant for large-scale operations. In addition, researchers are investigating the integration of CCS with renewable energy systems, which could enhance the overall sustainability of energy production.
Innovations in CCS are setting the stage for a transformative impact on how industries can balance their carbon footprints and operational needs.
Global Initiatives and Collaborations
Collaboration is vital for advancing CCS technologies. Various global initiatives are in place to promote research and deployment of CCS. For instance, the International Energy Agency (IEA) has created frameworks that facilitate knowledge sharing between nations pursuing similar goals. The keys to success in such collaborations include sharing best practices in technology, regulatory frameworks, and financing strategies.
Additionally, several countries have launched programs aimed at establishing CCS as a vital part of their climate action plans. The Global CCS Institute is another pivotal organization, working to enhance awareness and stimulate investment in CCS projects around the world. Their efforts connect stakeholders from government, industry, and academia, fostering an environment conducive to pioneering advancements.
Investments in pilot projects also provide a platform for learning and scaling CCS solutions. By testing innovative approaches in diverse contexts, stakeholders can gather empirical data and evidence needed for wider adoption. As these collaborations thrive, they help build public support and create a regulatory framework conducive to the growth of CCS.
The future outlook for CCS technologies is marked by potential. Effective strategies can reduce carbon emissions and contribute to climate goals. Continued innovation combined with strong international partnerships will be essential as we confront the pressing challenge of climate change.
Closure and Key Takeaways
In the discourse on climate change solutions, the role of Carbon Capture and Storage (CCS) technology emerges as a critical point of consideration. This article has navigated through various facets of CCS, elucidating not only its operational mechanics but also its implications for our environment. Understanding CCS is imperative for comprehending the multi-dimensional challenges posed by carbon emissions.
Through a detailed examination of the capture methods, transport logistics, and storage applications, the significance of this technology becomes clearer. CCS offers a pragmatic approach to reducing greenhouse gas emissions, particularly from fossil fuel combustion, which remains prevalent across global industries. However, it is essential to recognize that while CCS can mitigate carbon output, it does not wholly replace the necessity for renewable energy transitions.
"Carbon Capture and Storage is not a silver bullet, but a necessary component of a broader strategy to address climate change."
The summary of key insights presented throughout this article underscores the importance of innovation in CCS technology. The transition towards greener practices will largely depend on advancements in capture efficiency, effective transportation methods, and secure storage solutions. Additionally, understanding the economic viability of CCS implementation is vital for attracting investments.
The call to action for future research emphasizes the urgency of further inquiry into improving existing technologies and exploring new methods for carbon storage and utilization. Continuous research not only enhances our current capabilities but also informs policy-making, driving frameworks that will support widespread adoption of CCS initiatives.