Microalgae Reactors: Innovations and Their Impact
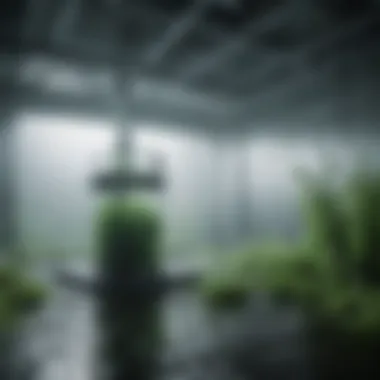
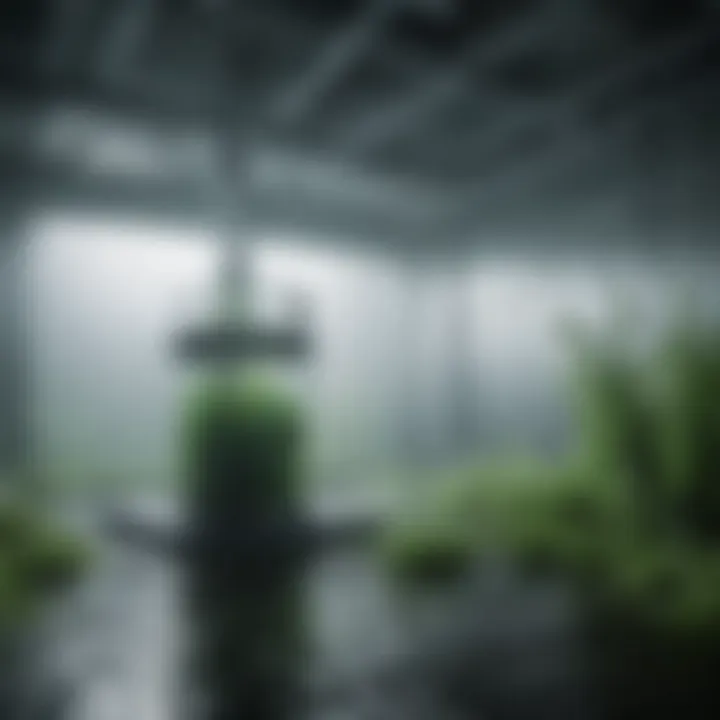
Intro
Microalgae reactors represent a convergence of innovative technology and environmental sustainability. As society increasingly grapples with challenges such as climate change, waste management, and energy demands, these bioreactors emerge as a critical solution. Microalgae are not only efficient in carbon dioxide fixation, but they also hold potential for valuable bioproducts, including biofuels, pharmaceuticals, and nutritional supplements. The aim of this article is to elucidate the advancements in microalgae reactors, analyzing their designs, functionalities, and applications across various fields.
The evolution of microalgae reactor technology has been significant. Enhanced designs have improved growth conditions, optimizing photosynthetic efficiency and biomass production. Key advancements in materials and engineering have also contributed to better overall performance. Therefore, understanding the essential parameters influencing microalgae growth is fundamental to leveraging their full potential.
This article delves into varied applications, ranging from biotechnology practices to renewable energy sources. It addresses both the challenges and opportunities presented by microalgae reactors, providing insights into their economic viability and environmental implications. The discussion caters to students, researchers, educators, and professionals, leading to a comprehensive understanding of this pivotal technology.
Research Highlights
Key Findings
Microalgae reactors have shown promising results in recent studies, highlighting their value in several sectors:
- Biotechnology: Enhanced strain selection and genetic engineering have improved yields of specific compounds.
- Waste Management: Microalgae can be used to treat wastewater, helping in nutrient recovery and pollution reduction.
- Renewable Energy: Biofuels derived from microalgae offer a sustainable alternative to fossil fuels.
These advancements indicate a growing recognition of the importance of microalgae in addressing global challenges.
Implications and Applications
The implications of these findings extend to diverse fields. In biotechnology, microalgae stand to revolutionize production processes for pharmaceuticals and food supplements by providing a greener, high-yield method of synthesis. For waste management, integrating microalgae into treatment systems offers low-cost, effective solutions for nutrient recovery, converting waste into valuable biomass. In renewable energy production, utilizing microalgae for biofuel generation presents a feasible pathway to reducing carbon emissions while simultaneously addressing energy needs.
Methodology Overview
Research Design
This article synthesizes data and findings from various research papers, governmental reports, and industry analyses. A comparative approach is employed to evaluate reactor designs and their performance metrics.
Experimental Procedures
Data collection involved meticulous examination of contemporary studies on microalgae reactors:
- Literature Review: Analysis of existing research provides insight into successful reactor designs.
- Case Studies: Highlighting real-world applications allows for a practical perspective on microalgae usage.
Through this method, a clear understanding of advancements and applications in microalgae reactors is established.
Prelims to Microalgae Reactors
Microalgae reactors represent a significant development in sustainable technology. Their ability to efficiently convert sunlight and nutrients into biomass makes them a focal point for innovation in various industries. In this section, we establish the foundation for understanding these reactors, their characteristics, and the multifaceted advantages they provide.
Microalgae are microscopic organisms capable of photosynthesis. They are found in diverse aquatic environments and possess unique properties that enable them to thrive in various conditions. The advancement of microalgae reactors facilitates the controlled cultivation of these organisms for diverse applications, such as biofuels, food production, and environmental remediation.
Definition and Overview
Microalgae reactors are systems specifically designed to cultivate microalgae under controlled conditions. These reactors can be classified into several types, including open pond systems, closed photobioreactors, and hybrid systems. Each design has its strengths and challenges, impacting the efficiency and suitability for specific applications. Main goals include optimizing light, temperature, and nutrient supply to maximize growth and productivity.
The significance of these reactors lies in their adaptability. They can be employed in a range of fields. These include agriculture, renewable energy, and biotechnology. The ability to tailor the cultivation environment supports the production of valuable bioactive compounds, thus making microalgae reactors vital in the contemporary quest for sustainable solutions.
Historical Context
The use of microalgae for various purposes stretches back several decades. Initially, research focused on microalgae's potential for food and feed supplements. As interest developed, scientific exploration expanded into biofuels and wastewater treatment. The first microalgae reactor designs were rudimentary, primarily based on natural ponds.
In the late 20th century, advancements in bioreactor technologies laid the groundwork for more sophisticated systems. Researchers gained a deeper understanding of microalgal biology, leading to optimized growth conditions. This shift marked a turning point in the development of microalgae reactors, paving the way for their modern applications in biotechnology and environmental management.
The evolution of these reactors can be seen as a direct response to the increasing demand for renewable resources and ecological sustainability. As the world faces pressing environmental challenges, microalgae reactors have emerged as a promising solution to mitigate climate change and reduce waste, aligning with global sustainability goals.
Biological Characteristics of Microalgae
The biological characteristics of microalgae are essential to understanding their applications and functioning in reactors. These microorganisms play a vital role in various biotechnological advancements due to their unique properties. Microalgae's ability to undergo photosynthesis allows them to convert sunlight and carbon dioxide into biomass and oxygen. This feature is central when considering their use in renewable energy applications, waste treatment, and even nutritional solutions.
Types of Microalgae
Microalgae are incredibly diverse, encompassing several species that vary in morphology, size, and habitat. Common types include:
- Chlorella: A unicellular green algae known for its high protein content.
- Spirulina: A cyanobacterium often marketed as a superfood due to its high density of nutrients.
- Dunaliella salina: Famous for producing high levels of beta-carotene.
- Scenedesmus: Known for its resistance to various environmental conditions.
Each type of microalgae exhibits distinct traits that influence their suitability for different applications in microalgae reactors. For example, Chlorella and Spirulina are widely used in producing dietary supplements, while Dunaliella salina finds applications in cosmetics and health food products.
Growth Conditions
The growth conditions of microalgae are crucial factors that affect biomass production and efficiency in reactors. These conditions encompass various environmental parameters:
- Light Intensity: Microalgae require light for photosynthesis, and insufficient light can limit growth. Optimizing light conditions can significantly enhance productivity.
- Temperature: Each species has an optimal temperature for growth. Deviations can lead to reduced growth rates or dormancy.
- pH Levels: The acidity or alkalinity of the medium influences nutrient availability and metabolic processes within the algae.
- Nutrient Availability: Nitrogen, phosphorus, and trace minerals are necessary for microalgae growth. Careful management of these nutrients ensures maximal growth rates and product yields.
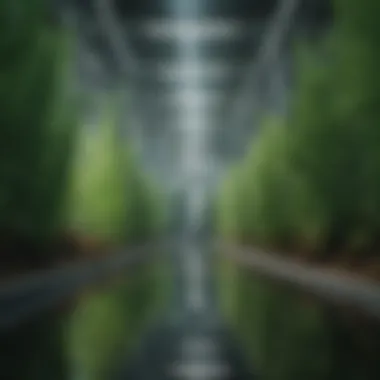
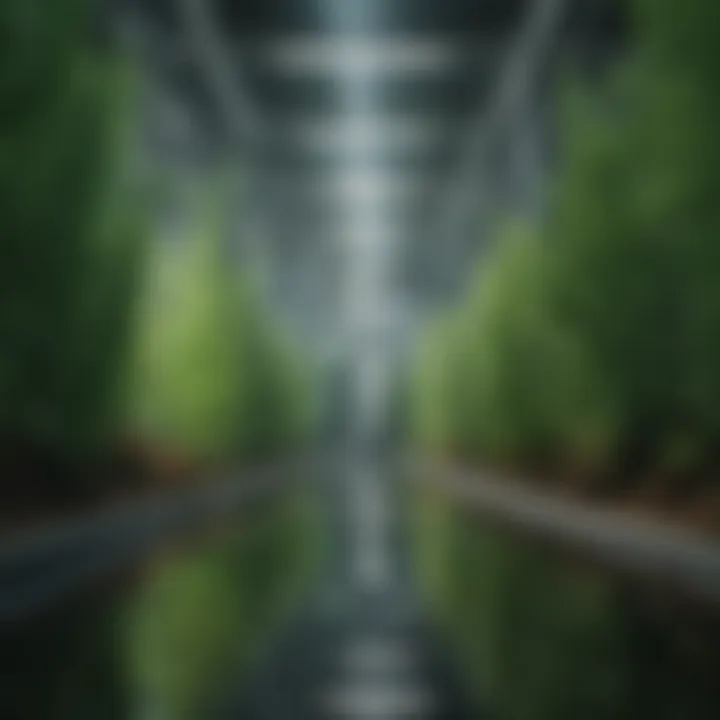
Understanding these growth conditions helps in designing more efficient reactors that cater to specific microalgae species, enhancing overall process efficiency.
Metabolic Pathways
Microalgae have complex metabolic pathways that allow them to utilize various substrates for growth. Their metabolism can be broadly classified into:
- Photosynthesis: The primary pathway where light energy is converted into chemical energy. This process results in the production of carbohydrates, which serve as energy sources.
- Respiration: A process that occurs in the absence of light, where microalgae oxidize carbohydrates to obtain energy.
- Lipid Accumulation: Many species can store excess carbon as lipids, which are crucial for biodiesel production. This pathway's efficiency can be influenced by growth conditions and nutrient availability.
By understanding these pathways, researchers can manipulate microalgae metabolism to optimize biomass production or enhance the synthesis of high-value products like omega-3 fatty acids and antioxidants.
"The metabolic capabilities of microalgae provide an extensive resource for biotechnological applications across multiple industries."
Reactor Design and Types
Reactor design is a fundamental aspect in the study of microalgae reactors. The choice of design significantly influences productivity, efficiency, and overall operational efficacy. Understanding the various reactor types allows researchers and industry professionals to select the most suitable system based on specific goals, whether these involve high biomass yield, effective nutrient utilization, or maximized light exposure. Each design comes with unique benefits and challenges, making it imperative to explore these features in depth. The right reactor system is not just about operational requirements; it often entails balancing economic viability with environmental impact, ultimately reflecting modern sustainability goals.
Open Pond Systems
Open pond systems are among the oldest methods for cultivating microalgae. These systems typically consist of shallow ponds or basins exposed to direct sunlight. They offer several advantages such as low capital investment, relatively simple design, and reduced operational costs. Additionally, the extensive area allows for significant biomass production under optimal environmental conditions.
However, open pond systems also face challenges. They are susceptible to fluctuations in temperature, contamination, and evaporation. Moreover, the lack of control over parameters like nutrient levels and pH makes achieving consistent production difficult. Nevertheless, they are often used in large-scale biofuel production where cost efficiency is prioritized over strict controllability.
Closed Photobioreactors
Closed photobioreactors provide a more controlled environment for growing microalgae. These systems consist of transparent tubes or vessels that allow light to penetrate while protecting the algae from external threats such as contamination and extreme weather. This design improves metabolic efficiency and enhances biomass yield because it allows for precise control of growth conditions, including temperature, light intensity, and nutrient availability.
Though they come with higher operational costs, closed photobioreactors can be economically viable depending on the application. They are particularly beneficial in producing high-value products like nutraceuticals, where purity and consistent quality are essential. The technology continues to advance, with innovations focusing on increasing efficiency and reducing costs, making these systems appealing for various commercial applications.
Hybrid Systems
Hybrid systems combine aspects of both open and closed designs. This approach aims to capitalize on the strengths of each system while mitigating their weaknesses. For instance, a hybrid system may utilize open ponds for preliminary growth phases, where lower costs are acceptable, then transition to a closed photobioreactor for final growth and harvesting.
The flexibility of hybrid systems enables better management of resources such as light and nutrients, potentially leading to higher overall productivity. However, their complexity demands more sophisticated management strategies and a thorough understanding of each subsystem’s dynamics. As research in this field progresses, hybrid systems are likely to play a significant role in enhancing the scalability and commercial viability of microalgae cultivation.
The effective design of reactors is crucial to maximizing the potential of microalgae in diverse applications, from renewable energy to bioremediation.
In summary, the design of microalgae reactors profoundly shapes their operational performance and applicability. Each system presents unique benefits and limitations, emphasizing the need for careful consideration in their selection and implementation.
Key Parameters of Reactor Performance
Understanding the key parameters of reactor performance is crucial for optimizing the efficiency of microalgae reactors. These parameters directly impact the growth and productivity of microalgae. Careful management of these elements is necessary to ensure that reactors operate at their best. Here, we focus on three primary parameters: temperature and pH balance, light availability, and nutrient supply. Each plays a significant role in the cultivation and viability of microalgae, which is integral to various applications.
Temperature and pH Balance
Temperature is a vital factor influencing microalgae growth. Each species of microalgae has a specific temperature range for optimal productivity. Extremes in temperature can inhibit growth or even lead to cell death. Generally, temperatures between 20°C and 30°C are favorable for many microalgal species.
The pH level of the culture medium is also crucial. Microalgae typically thrive in a slightly alkaline environment, with pH levels ranging from 7.5 to 9.5 being ideal for most species. Deviations from this range can lead to reduced photosynthetic activity and nutritional uptake. Therefore, maintaining a stable temperature and pH is essential for maximizing biomass yield and maintaining culture health.
It is essential to carefully monitor and adjust temperature and pH in microalgae reactors to ensure optimal growth conditions.
Light Availability
Light is perhaps the most important factor for photosynthetic organisms. Microalgae require sufficient light intensity to carry out photosynthesis, which in turn produces energy for growth. The depth of the reactor influences how light penetrates the culture medium, impacting overall efficiency.
There are several considerations regarding light availability:
- Intensity: High light intensity promotes growth but can also lead to photoinhibition, where excess light damages the cells.
- Quality: Light spectrum affects the efficiency of photosynthesis. Specific wavelengths are more effective for brown or green microalgae, which may necessitate the use of specialized lighting technologies in enclosed systems.
- Duration: The period of light exposure can range depending on the species cultivated. Daylight cycles can be manipulated to suit the specific needs of the microalgae being grown.
Nutrient Supply
Nutrients are required for the healthy growth of microalgae. Key macronutrients include nitrogen, phosphorus, and potassium, while micronutrients such as iron, zinc, and copper are also necessary in smaller quantities.
- Nitrogen Sources: Ammonium, nitrate, or urea can be utilized depending on the growth phase of the microalgae species.
- Phosphorus Availability: Phosphorus is critical for energy transfer within the cells and must be supplied adequately.
- Mineral Supplements: Micronutrients play various roles in metabolic processes, including enzyme function and nutrient assimilation.
A balanced nutrient supply, tailored to the specific needs of the microalgae, is key to maximizing biomass production and maintaining reactor stability.
In summary, careful management of temperature, pH, light availability, and nutrient supply are essential for optimizing the performance of microalgae reactors. A thorough understanding of these parameters can lead to better designs and operational practices, ultimately enhancing the potential applications of microalgae in various fields.
Technological Innovations in Microalgae Reactors
Technological innovations play a crucial role in enhancing the efficiency and sustainability of microalgae reactors. As the demand for renewable energy and biotechnological solutions rises, innovations will guide the advancement of microalgae utilization. These technologies not only optimize reactor design but also improve the harvesting and processing of microalgae products, making them more accessible for commercial applications.
Automation and control technologies are essential advancements in microalgae reactor systems. They enable precise monitoring and management of growth parameters, which is crucial for maximizing productivity. These systems facilitate real-time adjustments in light, temperature, and nutrient supply, allowing researchers and operators to respond swiftly to the changing conditions.
Nanotechnology also emerges as a significant player in reactor design. It offers potential enhancements in surface area and light absorption capabilities, which are crucial for effective microalgae cultivation.
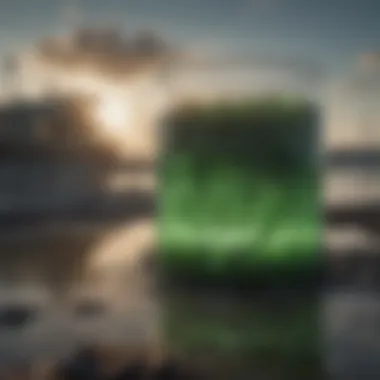
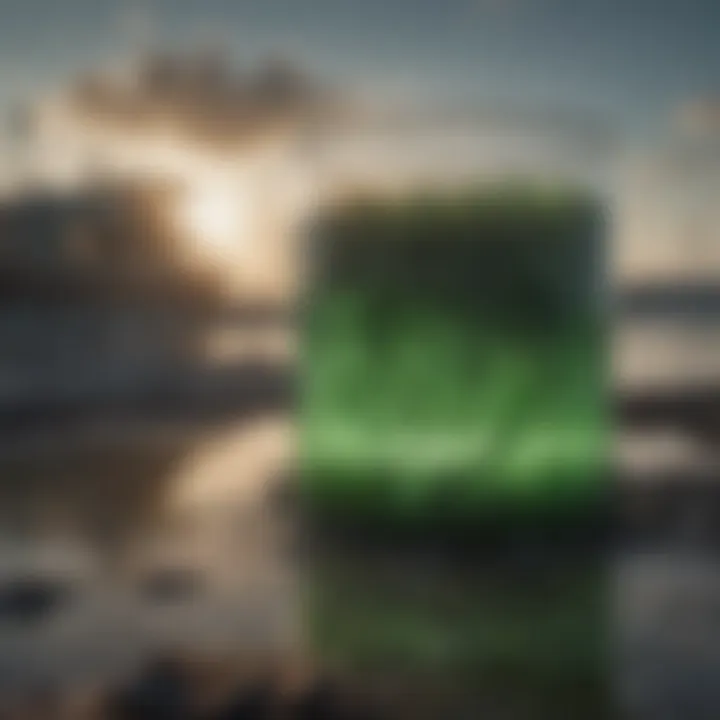
Automation and Control Systems
Automation and control systems significantly reduce labour costs and human error in microalgae production. These systems use sensors and software to automate monitoring and control processes, ensuring optimal conditions for microalgae growth. Through the integration of IoT (Internet of Things) technology, reactors can connect to centralized data systems, thus providing valuable data for decision-making.
One of the benefits of automation is the ability to collect continuous data on operational parameters such as pH, temperature, and light intensity. This data can enhance understanding of growth patterns and inform further research. Furthermore, automated systems allow for high-throughput experimentation, which accelerates innovation and development. For instance, autonomous nutrient dosing can be implemented, minimizing waste and ensuring the cultures receive precise nutrient concentrations.
Nanotechnology in Reactor Design
Nanotechnology in reactor design aims to improve efficiency and functionality through the application of nanoscale materials. These materials can significantly influence the light absorption properties of reactors. Enhanced light absorption directly correlates with increased photosynthetic activity in microalgae, which is vital for their growth.
By incorporating nanoparticles, it is possible to fabricate surfaces that enhance light diffusion or improve the delivery ofCO2 into the culture. Moreover, nanotechnology enables the development of advanced materials for constructing bioreactors, which can withstand corrosive environments and operate efficiently under various conditions.
The benefits of nanotechnology in microalgae reactors can lead to more sustainable practices in biofuel production and other microbial applications.
"Technological advancements are not just about innovation; they're about transforming possibilities into reality."
In summary, technological innovations, including automation and nanotechnology, are instrumental in shaping the future of microalgae reactors. These advancements ensure that microalgae production becomes more efficient, cost-effective, and broadly applicable. The continued exploration and implementation of these technologies will be vital as societies strive for sustainable solutions in energy, nutrition, and environmental restoration.
Applications of Microalgae Reactors
Microalgae reactors are increasingly recognized for their versatile applications across a range of fields. These reactors can contribute significantly to environmental sustainability, energy production, and food sources. Understanding the applications of microalgae is vital for optimizing their functions and addressing the challenges of modern society.
Bioremediation
Bioremediation is a crucial application of microalgae reactors. Microalgae can effectively remove pollutants from water and soil. They have a unique ability to absorb heavy metals, nutrients, and other toxic compounds. This characteristic makes them valuable in treating contaminants in wastewater.
The process involves cultivating specific microalgae strains known for their absorption capabilities. By integrating these systems, industries can lessen the environmental impacts of their operations. The implementation of bioremediation programs improves water quality while reducing costs associated with traditional methods of waste treatment. Moreover, the biomass generated can be further processed into valuable products, enhancing the overall efficacy of the treatment.
Biofuel Production
Biofuel production represents another significant application of microalgae reactors. With the pressing need for renewable energy sources, microalgae are emerging as a sustainable alternative. They can produce biofuels such as biodiesel, bioethanol, and biogas. Microalgae have high lipid content, which is essential for biodiesel production.
In biofuel reactors, favorable growth conditions are meticulously controlled to optimize lipid yield. These reactors can utilize carbon dioxide from industrial emissions as a nutrient source, thus playing a dual role: producing energy and mitigating greenhouse gas emissions.
The advantages of microalgae-based biofuels include:
- High productivity compared to traditional crops
- Minimal land usage
- Potential for carbon dioxide capture
Food and Nutraceuticals
Microalgae also play a vital role in the food sector, specifically in nutraceuticals and dietary supplements. Rich in proteins, vitamins, and omega-3 fatty acids, microalgae offer a nutritional profile that has gained attention from health-conscious consumers.
The cultivation of microalgae for food begins in reactors designed to ensure optimal growth conditions. Various species like Spirulina and Chlorella are popular in health products due to their health benefits.
Additionally, the growing popularity of plant-based diets has driven interest in microalgae as a protein source. Their ability to thrive in diverse environments makes them well-suited for sustainable food production. The potential market for microalgae in food and nutraceuticals is expanding, driven by consumer demand for health-boosting ingredients.
In summary, the applications of microalgae reactors are broad and impactful. From cleaning our environment to energy production and nutritional supplements, these systems have the potential to provide innovative solutions to pressing global challenges. Leveraging these capabilities could lead to significant advancements in sustainability and resource management.
Economic Feasibility of Microalgae Systems
Assessing the economic feasibility of microalgae reactor systems is crucial for their successful implementation. As studies highlight the potential of microalgae in contributing to sustainability, understanding the financial aspects helps stakeholders make informed decisions. A variety of factors determine this feasibility, including initial setup cost, operating expenses, and potential revenue streams.
The benefits of establishing microalgae systems extend beyond profit margins. They contribute significantly to waste management and renewable energy production, which can lessen long-term environmental liabilities. In this context, the economic evaluation must not only consider direct financial outcomes but also the societal and ecological advantages they provide.
Cost Analysis of Reactor Systems
Cost analysis of microalgae reactor systems involves multiple components. Initially, capital investment in the infrastructure is required, depending on the reactor type, whether it is an open pond system or a closed photobioreactor. Each type has varied setup costs. Closed systems may require more upfront investment due to their advanced technology and materials used for construction.
Operational costs are another key element to consider. These include:
- Energy consumption for maintaining optimal conditions
- Labor costs
- Nutrient supplies
- Water and wastewater management
In many cases, these systems can achieve economies of scale as production increases. This means that the costs per unit might decrease as more bioproducts are generated.
"Understanding the detailed cost structure is essential for making microalgae reactors economically viable. Without this analysis, stakeholders could underestimate the investments needed to maintain operations effectively."
Market Trends
Market trends indicate a growing demand for microalgae products. As industries are seeking sustainable solutions, the interest in biofuels, nutraceuticals, and food additives derived from microalgae is increasing. Current market dynamics show:
- A rise in government incentives for renewable energy production
- Increasing consumer awareness about sustainable food sources
- Expansion of research into new applications of microalgae, such as pharmaceuticals
This translates into favorable conditions for those considering investments in microalgae technology. Furthermore, technological improvements are likely to reduce production costs over time, enhancing market competitiveness.
Monitoring these market trends helps predict future opportunities and challenges for microalgae systems, as the technologies evolve to meet the inevitable shifts in demand and regulatory frameworks.
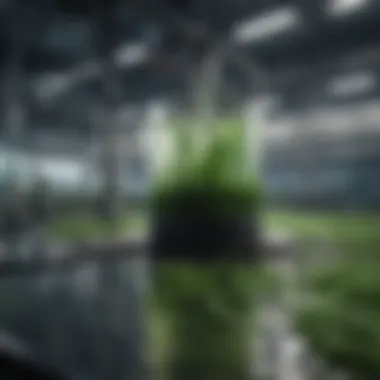
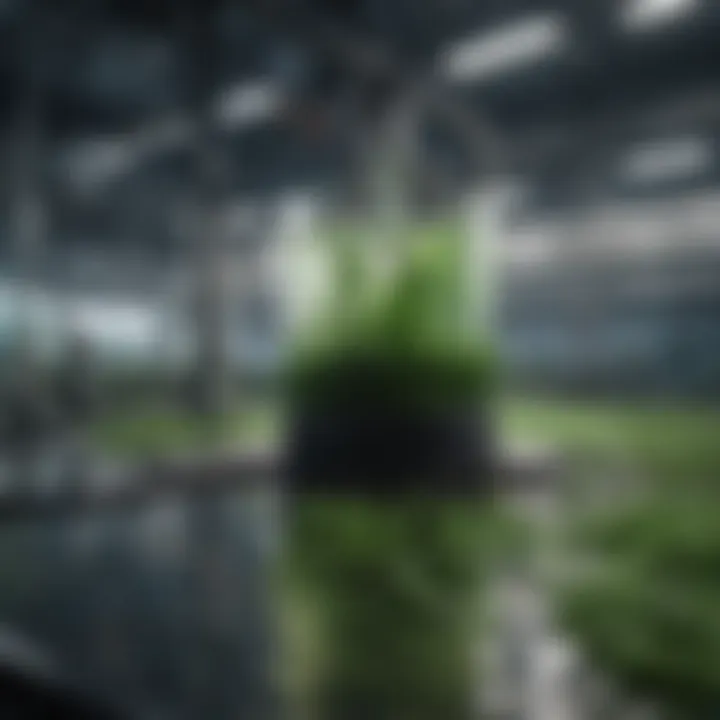
Environmental Impact
The environmental implications of microalgae reactors are a crucial part of understanding their overall value. These reactors play a significant role in addressing pressing ecological issues. They not only contribute to sustainable practices but also demonstrate potential for enhancing the planet's health.
Carbon Sequestration Potential
Microalgae are known for their high efficiency in carbon fixation. This process is essential, as it enables the capture and storage of carbon dioxide from the atmosphere. Microalgae utilize sunlight to convert carbon dioxide into biomass, which can significantly mitigate the effects of climate change.
Different studies indicate that numerous species of microalgae can absorb up to 10 times more carbon dioxide than terrestrial plants. By integrating microalgae reactors into urban and industrial settings, we can create systems that actively reduce greenhouse gas concentrations. Consequently, this can help governments and organizations meet carbon reduction goals and contribute to global sustainability efforts.
Some benefits of utilizing microalgae for carbon sequestration include:
- Enhanced biomass production.
- Potential for biofuel generation.
- Reduction in the overall carbon footprint of various industries.
Water Usage Efficiency
Water efficiency is another critical factor to consider when evaluating the environmental impact of microalgae reactors. Compared to traditional agriculture, microalgae require significantly lower amounts of water per unit of biomass produced. This characteristic becomes increasingly important in areas facing water scarcity.
Moreover, microalgae reactors can utilize wastewater as a nutrient source. This not only helps manage waste but also promotes a circular economy approach towards water usage. By employing these systems, industries can achieve more sustainable water management while producing valuable biomass at the same time.
According to research, certain microalgae species can grow using saline or wastewater, minimizing the need for freshwater resources.
In summary, the environmental impact of microalgae reactors encompasses both carbon sequestration and water usage efficiency. Understanding these aspects is vital for harnessing their full potential in addressing climate change and promoting sustainable practices within various industries.
Challenges and Limitations
In the realm of microalgae reactors, both challenges and limitations warrant substantial consideration. This topic is pivotal as it highlights the hurdles that researchers and industry professionals must navigate to optimize the use of microalgae for various applications. Understanding these challenges is essential for developing effective strategies to enhance reactor performance and broaden their utility across fields like renewable energy and bioremediation.
Scalability Issues
One of the pressing concerns in microalgae reactor systems is scalability. While small-scale reactors can achieve impressive results in controlled environments, transferring these successes to larger systems presents significant difficulties. The efficiency of microalgae growth often diminishes as the scale increases due to several factors:
- Light Distribution: In large reactors, achieving uniform light distribution is critical for ensuring that all algal cells receive adequate illumination. Insufficient light can lead to lower growth rates and productivity.
- Nutrient Management: As the volume of water increases, so does the complexity of nutrient delivery to microalgae. Ensuring that nutrients are evenly distributed can be challenging, impacting overall algal health and growth.
- Harvesting Challenges: Larger systems require efficient harvesting techniques to separate microalgae from the growth medium. This process can be resource-intensive and may undermine the overall economic viability.
Scaling up microalgae reactors thus requires innovative designs and technologies to maintain productivity while addressing these multifaceted issues.
Contamination Problems
Contamination is another significant limitation facing microalgae reactors. The presence of unwanted microorganisms can severely compromise the performance of these systems. Contamination can reduce algal yields, disrupt metabolic processes, and negatively impact the final product quality. Several factors contribute to this challenge:
- Competing Species: Bacteria, fungi, and other algae can rapidly proliferate, competing with the desired microalgae for nutrients and light. This competition can lead to lower overall yields.
- Environmental Conditions: Variations in temperature, pH, and nutrient levels can create favorable conditions for contaminants to thrive. Continuous monitoring and optimal control are necessary to mitigate these risks.
- Biosecurity Measures: Implementing effective biosecurity practices is essential to prevent contamination. However, these measures can add complexity and cost to reactor operations.
Success in mitigating contamination will depend on a combination of rigorous monitoring, improved reactor designs, and advanced sterilization techniques.
Future Directions in Microalgae Research
The exploration of future directions in microalgae research is essential for understanding the evolving landscape of this field. As global challenges grow, microalgae provide versatile solutions across various sectors. This section highlights emerging trends and the potential of genetic engineering in optimizing microalgae strains, addressing the future needs of industry and society alike.
Emerging Trends
Recent advancements in microalgae research highlight several key trends:
- Integration with Renewable Energy Sources: There is a significant push towards integrating microalgal systems with renewable energy systems, primarily solar and wind energy. This synergy optimizes resource use and reduces overall carbon footprints.
- Waste-to-Value Products: Emerging trends include utilizing agricultural and industrial waste as nutrient sources for microalgae growth, turning waste into valuable biomass. This not only promotes sustainability but also reduces environmental pollution.
- Multi-functional Applications: Research is shifting towards multifunctional applications of microalgae. They are increasingly seen as not just biofuel sources but also for products like biofertilizers, animal feed, and human supplements. This diversification provides economic resilience to microalgae production systems.
A growing body of research emphasizes the importance of scaling microalgae production to enhance efficiency and reduce costs, ultimately driving broader adoption.
This trend indicates a future where microalgae become integral to resource recovery and environmental management.
Potential for Genetic Engineering
Genetic engineering holds considerable potential for advancing microalgae applications. By manipulating specific genes, researchers can enhance desirable traits in microalgae strains. Here are some focal points regarding this potential:
- Increased Yield: Genetic modifications can lead to microalgae strains that grow faster and produce more biomass, making their cultivation more economically viable.
- Enhanced Metabolic Pathways: Tailoring metabolic pathways through genetic engineering can improve lipid production for biofuels or the production of other high-value compounds. This adaptability can meet the specific needs of different industries.
- Stress Resistance: Engineering stress-resistant strains enables microalgae to survive in various environmental conditions. This expands their potential habitats and application areas.
End and Summary of Findings
The exploration of microalgae reactors has illuminated their pivotal role in advancing biotechnology, environmental sustainability, and renewable energy. This article has dissected the complexities surrounding microalgae reactors, showcasing their design, functionality, and a spectrum of applications. Understanding these reactors is crucial, as they present solutions to significant global challenges such as waste management and energy production.
Microalgae reactors have garnered attention due to their efficiency and versatility. Their ability to produce biofuels, biodegradable plastics, and nutraceuticals positions them as valuable assets in transitioning to greener technologies. This summary encapsulates the importance of key insights drawn from the study, emphasizing their relevance in both academic and practical contexts.
Recap of Key Insights
- Diverse Applications: Microalgae reactors serve multiple sectors, including agriculture, pharmaceuticals, and energy. Their adaptability underlines the necessity of integrating them into various industrial processes.
- Technological Advancements: Innovations in reactor design, such as the implementation of automation and nanotechnology, enhance efficiency and scalability. These advancements make the reactors more viable for commercial applications.
- Environmental Benefits: The environmental impact of microalgae is substantial. They contribute to carbon sequestration and reduce the ecological footprint of waste disposal. This aspect is increasingly significant in light of global climate change initiatives.
- Challenges and Solutions: While the benefits are significant, challenges such as contamination and scalability remain. Addressing these issues through research and development is crucial for the viability of microalgae reactors.
Microalgae reactors not only promise efficient energy solutions but also represent a vital component in achieving sustainable environmental practices.
Implications for Future Research
Future research in microalgae reactors should focus on several critical areas:
- Genetic Engineering: Exploration into genetic modifications can lead to improved strains capable of enhanced growth and productivity, opening new avenues for industrial applications.
- Hybrid Systems: Investigating hybrid systems that combine the strengths of both open and closed reactors may yield higher efficiency and lower costs.
- Policy and Economic Analysis: A comprehensive analysis of policy frameworks and economic incentives could aid in the faster adoption of microalgae technology in various sectors.
- Public Awareness and Education: Increasing the understanding among the general public and stakeholders about the benefits of microalgae can drive support for research and application.